NEW !
Antenna Calculator NEW !
In most cases the design of a radio controlled clock receiver will determine
the dimensions of the required receiving aerial for the low frequency
band, because the prescribed dimensions must not be exceeded. Usually
the aerials work according to the principle of a magnetic dipole, their
size can be reduced largely by insertion of a ferromagnetic material.
Compared to the antennas for reception of the electrical field the magnetical
aerials have the advantage that - regarding the frequency range of interest
- they can be easily matched to the input circuit of the receiver. By
completion with a parallel-connected capacitor a parallel resonant circuit
is originated, which can be tuned to resonance and which allows to adjust
noise or impedance matching. Herewith the voltage of the antenna input
circuit, which is induced in the antenna coil by the electromagnetic field
of the transmitter, is increased with the quality factor Q. It is presumed,
that the input impedance of the receiver is high - like that of the integrated
circuits T4223B and CME6005 manufactured by ATMEL Germany.
The terminal voltage of the ferrite aerial is determined by several parameters.
It can be calculated with the following formula:
(1) |
 |
with:
UA = terminal voltage
Q = circuit quality factor
Df = 3 dB bandwidth
µeff = effective permeability
n = number of turns
A = cross-sectional area of the conductor loops
E = electrical field strength at the receiving position
α = angle of the magnetic field referred to the axis of the aerial
The discussion of formula (1) shows, that:
- the electromagnetic field of the transmitter at the receiving position
is important
- a directional effect exists
- the induced voltage is higher with thicker ferrite rods
- the ferromagnetic material has an influence
- high numbers of turns lead to high antenna voltages
- a high quality factor increases the antenna voltage
Additionally it should be mentioned that the selectivity
of the antenna circuit increases with a raising quality factor Q,
which improves the noise immunity regarding reception on
adjacent frequencies due to reducing the bandwidth.
For the practical dimensioning of the antenna input circuit it is bear
in mind that the different parameters partly are depending on each other.
On the one hand a higher number of turns or a increased cross-sectional
area effects a higher inductance, on the other hand - provided that the
diameter of the wire is the same - it leads to a larger ohmic resistance.
With a raising number of turns the antenna voltage grows, but the also
increased ohmic resistance counteracts against the raising of the quality
factor (see formula 5). Further the effective permeability depends of
the realisation of the winding, as the placement on the ferrite rod and
the distance from the core. That means that the realisation of the
aerial must be optimised for every application (see also section
"practical antenna design "). Concerning the directivity, this
is an unwanted effect especially for applications, which can't be turned
or moved.
For those applications special antenna designs have to be used, which
provide an elliptical or omni directional receiving characteristic. The
latter can only be achieved with rod aerials. More detailed explanations
can be found in the relevant patent literature e.g. DE 3504 660 C2, DE
37 01 378 C2.
Generally it is to state, that - to consider the worst case - noise
matching should be the target, due to the low field strength's
that must be expected. Thus the most essential criteria for the electrical
dimensioning of the antenna is fixed. The optimal generator resistance
of the input circuit, for a tuned antenna this is their resonant resistance,
is approximately 180kΩ to 300kΩ for the high resistance receiver
T4223 / U4223 and about 50 kΩ to 100 kΩ for the CME6005.
The optimal value is not easy to determine, as the matching curve shape
is rather flat. This means that a rather large range for the nearly optimal
resonant resistance can be permitted.
In the frequency range of interest the required resonant resistance can
be realised by adequate dimensioning of the antenna circuit. For two reasons
it has turned out to be suitable to set the dimensioning to the low-resistive
range of the noise-matching curve. Firstly the required quality factors
are easier to achieve and secondly the feedback is reduced.
|
1.1. Calculating of the antenna input circuit
as an example for U4223 |
|
|
|
|
The input impedance of the integrated circuit is neglected. It is high
compared to the required generator resistance for optimal noise matching.
To reduce feedback the resonant resistance of the antenna circuit shall
be set to Rres = 180 kΩ for the
U4223.
A quality factor of 100 is realistic and can be achieved without special
efforts. The circuit capacitance can be calculated for f= 77,5 kHz (German
DCF) as follows (example for the 180 kΩ Antenna):
(2) |
 |
The standard value close to it is: C = 1,2 nF
The corresponding circuit inductance can be found with:
(3) |
 |
The bandwidth is calculated with:
(4) |
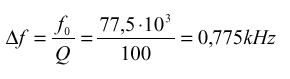 |
If the loss resistance of the capacitor is neglected, the following formula
is valid:
(5) |
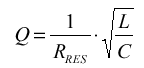 |
Due to the very small bandwidth of the input circuit exact temperature
compensation is essential. For the same reason the aerial must be tuned
to resonance with the received time signal transmitter at the actual mounting
position. Please note that e.g. metallic objects close to the antenna
will influence the alignment and may lead to the necessity of corrections.
Formula (2) can also be used to calculate the resonant resistance Rres
if the capacitance is known and the bandwidth Δf is measured. To
avoid any influence of components used for coupling between signal generator
and antenna, the signal generator should be equipped with a loop antenna
for this measurement. The probe that is used to measure the antenna voltage
should be an active one. Its resistance must be high compared to the resonant
resistance of the antenna and its capacitance must be very small compared
to the capacitance of the antenna circuit, so that neither the quality
factor nor the resonance frequency of the antenna are influenced in a
significant way.
This calculation can also be done for the CME6005 with the 40 to 100
kΩ Antenna resonant resistance.
The input stage of the integrated receiver circuit is designed as a differential
amplifier. The input transistors must be biased externally in case of
the U4223, the CME6005 has in build biasing resistors of 200 kΩ.
In the case of U4223 three different input circuits are possible, as figured
out in the following circuit diagrams. Please note: the CME6005
can only use a single ended antenna, because of it's in-build biasing
resistors (Fig. 1 d).
A balanced connection of the aerial offers the following benefits for
the U4223:
- high in-phase suppression
- lower sensitivity against static irradiation
- lower unwanted feedback and therefore less critical construction
A single-ended antenna requires less expenditure.
Different connections of the aerial
|
|
a) single ended |
b) balanced by
means of a cetnre-tapped coil |
|
|
c) balanced by
means of two resistors (2,2MΩ proposed |
d) single ended
antenna |
For the U4223 the variation c) is proposed because of the benefits of
a balanced connection, while the expenditure for a tapped coil is avoided.
The two resistors used to bias the input transistors should be high resistive
to conserve the quality factor of the antenna.
Also the ferrite material has an influence to the quality of the antenna.
The user is hold to select a material with excellent behaviour in the
frequency range from 20 to 120 kHz in order to reduce losses from the
ferrite material. (See also section practical
antenna design)
|
1.4. Comparison of the achieved sensitivity
with different ferrite antennas |
|
|
|
|
For the following comparison different aerials were connected to a demo
board of the U4223. An RF generator was modulated with the time signals
according to the coding of the German DCF77 transmitter. The generator
output was fed to a loop antenna, while the investigated receiving antennas
were placed in the axis of the loop antenna at a defined distance. The
receiver boards were connected with a data processing unit to decode the
time information. The sensitivity was defined by the correct decoding
of at least two data telegrams out of four. All measurements were carried
out in a shielded room (faraday cage) to avoid failures caused by external
disturbing sources. Nevertheless the reproducibility of these measurements
is critical. Field distortions inside the shielded room may have an influence;
therefore the following results should be regarded only as a rough guideline
for own antenna developments. The sensitivities are referred to antenna
No. 1. The antennas designed for the use with U4223.
antenna 1 |
diameter oft the ferrite rod: 10 mm, length: 30 mm
C = 830 pF, unloaded Q = 100, resonant resistance Rres = 250 kΩ
Connecting according to figure 1b)
Relative sensitivity: 0 dB |
antenna 2 |
diameter oft the ferrite rod: 10 mm, length: 30 mm
C = 5,6 nF, unloaded Q = 65, resonant resistance Rres = 24 kΩ
Connecting according to figure 1c)
Relative sensitivity: + 3 dB |
antenna 3 |
diameter oft the ferrite rod: 10 mm, length: 30 mm
C = 1,2 nF, unloaded Q = 100, resonant resistance Rres = 170 kΩ
Connecting according to figure 1b)
Relative sensitivity: + 5 dB |
antenna 4 |
diameter oft the ferrite rod: 8 mm, length: 65 mm
C = 720 pF, unloaded Q = 95, resonant resistance Rres = 270 kΩ
Connecting according to figure 1c)
Relative sensitivity: + 5 dB |
antenna 5 |
diameter oft the ferrite rod: 8 mm, length: 65 mm
C = 1,35 nF, unloaded Q = 115, resonant resistance Rres = 175 kΩ
Connecting according to figure 1c)
Relative sensitivity: + 6 dB |
It can be clearly seen, that the antenna can increase the system sensitivity
significantly if the antenna is designed in the right way. More increase
of the sensitivity of the system can be achieved by using different diameters
of wire or by using multiwire wires.
|
1.5. Receiving direction of root antennas |
|
|
|
|
The antennas useful for time code reception, are ferrite-root antennas.
This antennas have always a bi-directional receiving behaviour like a
classical dipole antenna. (see Figure) By changing the diameter and the
length of the root, the characteristic of the reception behaviour can
be influenced somewhat. A bigger diameter and a shorter length leads to
a less strong bi-directional reception. This information should be taken
into consideration by planing the antenna together with the part in which
the antenna should work.
|
|
Root with short length and bigger
diameter |
Root with long length and thin
diameter |
|
2. Practical antenna design |
|
|
|
|
Step 1
The CME6005 is designed to be used with a simply antenna, following
the Figure 1d).
The resonant resistance Rres should
be in the range of 40 to 100 kΩ. Out of the formula
(5) we can calculate
the resonant resistance Rres
Essential for the Rres is the quality
factor Q and the L/C ratio.
To avoid a lot of complicated calculations, we have to use some measurements.
The quality factor Q can be found by measuring the voltage oft the resonant
frequency from the antenna and the both corner frequencies with 3 dB (0,707
times) less voltages. This both corner-frequencies give us the bandwidth
(3 dB bandwidth = Δf ).
For example: we have the resonant frequency of DCF77 =
77500Hz and a bandwidth of 775 Hz, then we use the simple formula coming
from (4):
In this case the Q-factor is 100
Step 2
To find the values for the inductance and the capacitance we have to
use the formula for a resonant circuit:
Also here we chose a practical value for the capacitance.
The value are mainly between 2,2 nF and 10 nF. The loss factor of the
capacitance has to be low (0,0005 tanδ) Out of this assumption we
can calculate the value of the inductivity out of (3):
Step 3
Now we need to choose the ferrite material in order to calculate our
windings.
The ferrite should have a permeability (µ) of 2000 (real values
can differ, also 1800 is ok) and has to be good for frequencies from about
20 to 120kHz to avoid losses in the ferrite material itself. We have also
to take in account the temperature factor of the ferrite. This is not
a big issue with low quality factors (~50) but with quality factors of
100 and up we have to care, that with changing temperature the resonant
frequency of our tuned antenna will not move, because the bandwidth of
a antenna with high quality factor (means a good antenna) is small compared
with low Q-factors.
Step 4
We need now to know the effective permeability (µeff)
of the ferrite material. If we cannot get it from the data's from the
supplier, we can get the value easy by putting 100 windings onto the ferrite
and than testing the inductance. The following formula shows the way how
to get this factor:
The inductance is calculated by: |
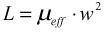 |
L= Inductance µeff
= effective permeability
w = number of windings |
If we calculate with 100 windings |
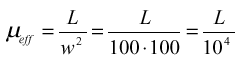 |
To calculate the necessary windings we use: |
 |
Step 5
The wire material and the type of doing the windings have an influence
to the quality of the antenna.
If we have no limitation of the area where we can bring up the windings,
the diameter should be 0,28 mm.
If we have a limitation of the area for windings the whole possible area
has to be used in order to decrease the wire diameter not below 0,15 mm.
From formula (5) can be seen that
a reducing of the antenna capacitor leads to a higher necessary inductance
this will increase the effective antenna voltage and also the resonant
resistance. The higher necessary windings on the ferrite lead to more
losses due to spread capacities between the windings and therefore a decreasing
of the quality factor Q. If there is a necessity to use a high number
of turns, the reducing of the thickness of the isolation of the wire can
be taken in account. The same number of windings and the same diameter
of the wire can lead to different Q factors if the isolation and the accuracy
of the windings are changed.
Step 6
In all cases, the antenna has to be tuned to the desired frequency, because
the ferrite itself as well as the capacitor have some tolerances. The
easiest way is to make the coil movable on the ferrite. The capacitor
has it's best place on the windings or as close as possible to the coil.
A phase-gain analyser is the ideal tool to very quickly find the resonant
point. Now moving the coil on the ferrite varies the resonant frequency
to the desired value e.g. 77500kHz (DCF77).
This test can also be done according the description in the datasheet.
In this case the capacitance of the test-probe has to be taken in account.
This capacitance detunes the antenna.
A more difficult way is to measure the antenna in conjunction with an
application board of the CME6005. The voltage at the capacitor PK
is a value of the input-voltage from the antenna. By measuring the voltage
at this point, the antenna can be optimised. Essential is that this is
done in a faraday cage to avoid any influence from other carriers. This
test point (PK) shows the sum of all frequencies the antenna catches.
It is easy to understand that each distortion leads to a wrong result.
A variation of the L/C of the antenna can lead also to more optimized
results.
If all the above mentioned points are taken into account, it is still
good, to do some variations of the antenna in order to find the antenna
with the best result in the specific application.
For the most important frequencies the following dimensioning results
in usable antennas, but not optimised to a specific application.
For the U4223: |
|
|
|
fo =
77,5 kHz: |
C = 1,2 nF, |
L = 3,50 mH |
|
fo =
60,0 kHz: |
C = 1,5 nF, |
L = 4,80 mH |
|
fo =
40,0 kHz: |
C = 2,2 nF, |
L = 7,20 mH |
For the CME6005: |
|
|
|
fo =
77,5 kHz: |
C = 2,2 nF, |
L = 1,92 mH |
|
fo =
60,0 kHz: |
C = 4,7 nF, |
L = 1,50 mH |
|
fo =
40,0 kHz: |
C = 6,8 nF, |
L = 2,33 mH |
The quality factor this dimensioning was based on can be achieved only
with high-quality capacitors.
To avoid feedback, the connecting wires to the aerial should be shielded
or at least twisted.
|
4. General hints for troubleshooting |
|
|
|
|
The antenna is mainly sensitive to the magnetic component of the transmitted
frequency. There are a lot of possible external and internal electrical
and magnetically distortions. Thus, close to the antenna any wire with
AC components on it has to be avoided. This is also valid for the output
of the receiver. The receiver is a direct receiver and components from
the output signal, coupled to the antenna by electrical field or parasitic
capacitors, cause a reduction of the sensitivity. The leads of the antenna
has to be shielded or at least twisted to avoid unsymmetrical induction
of noise. Also metal component e.g. batteries, holder for batteries, detune
the antenna and reduce the sensitivity of the system. LCD display have
a extensive field of distortion. This components have to be placed as
fare as possible from the antenna AND receiver.
Special attention has to be given to micro-controller. They produce a
high noise level with high slope rates. These high slope rates can interfere
to the antenna and lead to a short ringing of the antenna, this results
in a sensitivity decrease of the system.
The low pass filter of the Vcc for the micro-controller is also a point
which creates noise.
Summarisation:
- Place antenna far away from electronic components which create interference
e.g. processor, display, signal lines....
- Place antenna minimum 20 mm away from metallic parts e.g. batteries
- If you measure at U4223, pin REC, INT or DEC you must use a test probe
with impedance higher than 1 GΩ. This is also good for testing CME6005
pin PK
- Use separate power supply lines for receiver, processor and display.
- Use RC combination to block any distortion on Vcc.
- Use only one central ground point for the receiver.
- Make short connection from the crystals to the receiver (avoid additional
wire capacity)
- Avoid any current loops as well as ground loops nearby the IC.
- Provide shielding under the IC with ground layer of PCB if possible.
|
5.Software decoding Hints |
|
|
|
|
The time code signal generally has the following format:
Each second is 1 bit transmitted. The place of the bit (1 to 59) gives
the meaning of the information of this bit.
Example:
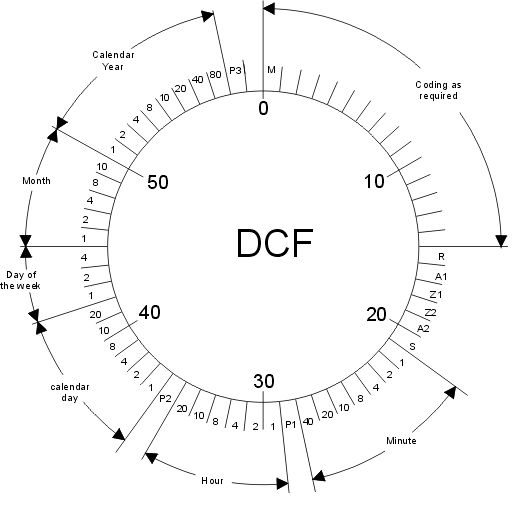
The signal at the antenna looks like that:

To decode a Time-Code-Signal several principal steps are necessary.

To do the information-decoding, the coding of the transmitter used has
to be taken. Examples can be taken out of the datasheet.
Please note: The user is responsible to verify the information from
the transmitters. If the coding changes, no warning is given from the
submitter of the paper.

|